J.Dodd, J. Benco
ABSTRACT
Due to the lack of exposed and sampled outcrop the late Cenozoic Pleistocene – Pliocene transition has become mostly known through drilling, seismic surveys, and well logging techniques. The AND-1B Antarctic core has produced 1285m of usable core, oldest being early Miocene to the youngest as Pleistocene1. This core provides the most thorough record of Antarctic ice-sheet dynamics, and climate interactions. Noted interval of this core is about 90m thick warm Pliocene diatomite, which has plenty of potent possible samples of diatoms from this section of core1. Diatoms from this core and their subsequent δ18O values can be used as a proxy for paleoclimate2. Previous research on the AND-1B Antarctic core has yielded diatom δ18O for major expansion of the ice sheet of the Ross sea at ~3.3 Ma and sea temperature cooling at ~2.5 Ma2. Most studies from the AND-1B core have focused primarily on the Early to Mid Pliocene and prior. This is mostly due to the abundance of sample opportunities as well a the “cleanliness” of the sample diatoms. This study is focused on the relatively thin units of diatomite within the Late Pliocene to Early Pleistocene to highlight that transition. Focusing on Late Pliocene to Early Pleistocene transition (89.95-80.61 meters below sea floor), using SEM (Scanning Electron Microscope) descriptions, and bulk elemental analysis EDS (Energy-Dispersive X-ray Spectroscopy) of the processed core samples, inferences can be made as to the paleo-environment for this cooling transition.
Historical Overview
The AND-1B Antarctic core, gathered subsea sediment beneath the McMurdo Ice Shelf (MIS)1. The AND-1B drill site is located at southern McMurdo Sound at 77.89˚S, 167.09˚E, and recovered from about ~850 m of water depth4. The McMurdo Ice Shelf forms the Northwest part of the Ross Ice Shelf. Sediment collected from the AND-1B core contains glacimarine and volcanic sediment in the Windless Bight region of the MIS1.
During the warmest intervals of the Pliocene (4.0-3.5 Ma) recorded an average global temperature about ~2-3˚C warmer than current temperatures2. During this period the WAIS (West Antarctic Ice Sheet) was greatly reduced. Due to global temperature spike, the average global sea level was about 5-40m above current levels2.
That situation transitioned into a cooling period about ~2.7Ma1. This change to a cooling situation has been attributed to (but not conclusively) declining pCO2, changing Milankovich cycles (Earth orbital geometries), possible tectonic influences, increased ocean stratification, precipitation in the northern latitudes, reduced zonal sea-surface temperatures gradients in the equatorial Pacific Ocean2. Due to this large number of factors, the role of Antarctica in Late Pliocene to global cooling is ambiguous. For during this time the expansion of the WAIS marine ice sheet has disrupted the Southern Ocean Circulation2. This increase in Antarctic marine ice sheet formation implies, Antarctic Sea cooling, and change in diatom facies.
Tectonic Setting
Regional volcanics originate from Victoria Land Basin (VLB). VLB is a ~350 km long, half graben rotated to its western side1. Regional rifted has occurred near the VLB since the early Eocene4. This rifting basin has accommodated about 10km of glacimarine sediment interbedded with volcanic stratigraphic units1. The crustal thinning during the Oligocene synrift phase has caused pronounced subsidence, which was slower and thermally controlled in the early Miocene1. The most recent period of rifting started in the center of the VLB during the Late Miocene and is actively rifting today1. Ross Island volcanism has contributed much to recent subsidence of the area, allowing for a large increase in accommodation space. The terror rift was formed in this fashion and has accommodated ~3km of Neogene sediment beneath the Windless Bight region1. This creation of accommodation space has allowed both extensive glacimarine sediment accumulation as well as during interglacial cycles, deep open water which allows for greater sedimentation of a larger variety of biogenic silica organisms.
Ice Flow and WAIS Sheet Dynamics in AND-1B Drilling Site
This area is directly affected by the advancement and retreat of the West Antarctic Ice Sheet (WAIS)6. The advancement and retreat are in correlation to the nearby influences of the EAIS, however the fluctuations of the WAIS are not stable and vary drastically6. Throughout the past ~3Ma the WAIS has experienced smooth transitions into large ice sheet volumes with extreme collapse due to “super-interglacials” at this time6. According to Pollard, the AND-1B drill site correlates to drill sites in the Amundsen, and Wendell sea6. Treating this section model as “one-dimensional” gives confidence that the values of the AND-1B core represents the overall advancement and retreat of the WAIS as a whole6.
Early/Mid Pliocene Stratigraphy and Ice Sheet Dynamics
During the Early Pliocene (586.45-146.79 mbsf) the AND-1B has distinct massive diamictite units which are represent subglacial to grounding zone, covered by stratified diamictite units, with local mudstone and sandstone with dispersed clasts, which are glacimarine advancement facies1. These move to massive or stratified diatomite, which is interpreted as open ocean/ice free settings. These cycles are interpreted as large oscillations of the dynamic ice sheet both in volume of the ice sheet and extent into the Ross Sea1. The transitions from diamictite to diatomite are quite sharp indicating a rapid transition between glacial to interglacial cycles5. The lower 10m of diatomite of this interval is unique in this section as it contains lonestones and gravity flows, which indicate that the ice sheet was nearby and quite stable for some time before retreating during the Mid Pliocene Warmest Period (MPWP)5. During the Mid Pliocene (289.00-146.79 mbsf), was the warmest time of this period, containing a large ~90m thick diatomite layer, which indicates high productivity and an open ocean setting2. This implies a large section of the WAIS (West Antarctic Ice Sheet) was locally absent and did not cover the region for at least one Milankovich cycle5.
Late Pliocene/Early Pleistocene Stratigraphy of AND-1B
The AND-1B core spans about 60 glacimarine cycles, due most likely to Milakovich related cycles1. Typical units have been alternating diamictite and diatomite layers, with known and dated volcanic sandstones, and mudstones1. The Pliocene-Pleistocene transition spans about (146.79-82.72 mbsf). 146.79-134.48 msbf in this section of the core represents a glacial-interglacial cycle; for directly above lies a diamictite which is primarily comprised of basaltic sandstone1.
134.48-120.00 mbsf is comprised of volcanic mudstone and sandstone1. This section also houses a volcanic breccia with 40Ar/39Ar ages , dating to about 1.6 Ma1.
Transition into a large diamictite layer, directly above (109.7-97.2 mbsf) signals a return of a marine ice sheet above this core1. This section however, has about ~.4Ma of missing section, from about 1.5-1.1 Ma1. This period of missing time is thought to have about 2 Glacial cycles missing from the section1.
97.2-86.60 mbsf contains the youngest biosilaceous sediments of the AND-1B core1. This is interbedded with volcanoclastic sediments as well as biogenic silica. Which represents an open water condition with biological productivity as well as reworked turbulent water conditions2. This would imply that there was no sea ice beyond the calving line in this area1. Which marks the beginning of the stable glacial cyclicity and colder settings leading up to modern time1.
Directly overlying this Diatomite section is a layer of lapulli tuff which has been 40Ar/39Ar dated from sanidine phenocryst to 1.01 Ma1. This section of core is the target of our project as this transition into glacial cycles of 80-120ka represent the “modern” time-scale of glaciation and a dramatic shift from the variable glacial cyclicity of the Pliocene. This particular transition is correlated with the Jaramillo Subchron1. Which is correlated to the abnormally warm period of this transition, named the “super-interglacial” of Marine Isotope Stage 311.
Diatom Species and Implications
The biostratigraphy of this region has added to the age constraints of the dateable volcaniclastics in this area. Specifically from the Pleistocene-Pliocene transition (146.79-82.72 mbsf) three distinct diatom species have been collected within those diatomite layers4. Two species being in the concise section of core for this study (89.80-86.60 mbsf). Actinocyclus ingens Rattray and Thalassiosira torokina Brady4. Both of these species are the diatoms that have been collected at 86.90 mbsf in the AND-1B drill site, and produced the frustules that are being analyzed in this study. These species are key as to water conditions at that time. Actinocyclus ingens Rattray is a quite fickle diatom. As that species survives at a water depth of 150-220 m and a water temperature of -0.128˚C to 1.08˚C4. Making these quite deep photic diatoms in comparison to near surface relatives. Thalassiosira torokina Brady can live in a variety of water depths as long as the water temperature is similar to Actinocyclus ingens4. Typically the extants of Thalassiosira torokina Brady are relatively near surface (0-100m water depth), which would imply that this species at that time would also have a near surface presence4. These two species give rise to the notion that at this time there was open water conditions with good visibility to the lower levels of the photic zone. This also implies that this “super-interglacial” during the Pliocene-Pleistocene transition the amount of nutrients, and nutrient gradient of the Ross Sea at this time were incredibly high4. This poses a problem for gathering δ18O values from this section of core, as both diatom species may be representing two different oxygen sources. The slightly deeper Actinocyclus could be in equilibrium with the seawater, while the Thalassiosira might be representing the mixing of glacial meltwater and the saline seawater, which would pull the δ18O positive as there would be an influx of 16O rich glacial meltwater.
Diatom Frustule δ18O Values and Relationship to Paleoclimate
δ18O values are the ratio of 18O/16O of the sample to the international standard, Vienna Mean Sea Ocean Water (VSMOW)8. As diatoms are single celled, photosynthetic organisms that produce SiO2 shells that form in equilibrium with the water, the δ18O value of these frustules can be used as a proxy for the δ18O of the mean ocean water8. The δ18O value of diatom frustules represent the equilibrium δ18O value of the seawater of formation8. This area is unique as it is relatively close to the continent and not in an open ocean setting, other factors may be affecting the values for these frustule δ18O values. If the waters of the Ross sea are well mixed, the δ18O values would be indicative of the bulk ocean and would correlate to the δ18O values of the benthic stack (Liesecki and Raymo).
As this site is located close to the coast it is possible to have sea brines affecting the δ18O values in this region. If that was the case, it would likely be that as sea brines are enriched in the 18O that a more negative δ18O signal would be produced8. These brines could start as saline differentiation of the local water with due to salinity gradients, or due to groundwater of the continent with collection of dissolved ions8. This groundwater brine factor seems to have a grounding in the previous sections of the core. Tirzah and Dodd analysis on δ18O of deeper sections of the core have seen about ~10ka lag between the δ18O values of the diatoms and the benthic stack values (Liesecki and Raymo)8.
If the opposite occurs in these samples it might be the case that during this time freshwater pulses from the ice sheet would be dominant in the photic region artificially shifting the δ18O values in a positive direction. If this was the case then the groundwater would be less effective than freshwater pulses6. According to Pollard et.al analysis of the AND-1B core from the Mid Pliocene, there is a correlation between δ18O values of deep-sea-core diatoms and sub-ice melts in the region6.
If the assumption that this region at this time is not in equilibrium with the bulk ocean water, then the δ18O values would represent if this environment was groundwater/brine controlled or freshwater pulse controlled.
If the assumption that this region represents well-mixed water, then the δ18O would represent the amount of freshwater in the form of ice that is present at the Pliocene-Pleistocene transition.
Hypothesis
Due to the lag time of about 10ka between the already documented δ18O from previous Pliocene and Miocene diatoms as compared to the benthic stack, there seems to be a further factor affecting the δ18O values of the diatoms from the AND-1B core. Using Scanning Electron Microscope and EDS bulk elemental analysis of processed diatom samples post-heavy liquid separation can provide evidence as to the natural controls of deposition and source of oxygen for frustule equilibrium in the AND-1B drill site.
Materials and Methods
The procedure to prepare our samples for analysis followed Henderson’s Preparation of lake sediments for diatom oxygen-isotope analysis9. This includes a 4 stage procedure with the stages being: 1. Organic and carbonate removal (H2O2, & HCl), 2. Additional cleaning (HNO3), 3. Sieving at 100μm , 50 μm, 25 μm, 10 μm and 5 μm , 4. Heavy liquid separation (sodium polytungstate)9.
As this section of core is relatively shallow as compared to its Miocene and Pliocene counterparts, and bounded by volcanic lapulli tuff and diamictite, we expected a large amount of organic matter and not much carbonate within the samples.
Stage 1. Consisted of powdering the core samples with a mortar and pestle and adding 5mL of 30% concentration H2O2 and allowing for reaction. These samples were then heated in order to accelerate the reaction between the organic matter and hydrogen peroxide. Once the reaction was completed, the byproducts were neutralized with the addition of 50% HCl and centrifuged at 2000 rpm for 20 minutes. Post centrifuge, the liquids were decanted off, and filled with water. These tubes were then centrifuged at 1200 rpm for 20 minutes and decanted. This process occurred three times of distillation and decanting. Once completed 5% concentration HCl filled the sample tubes and were left for 12hr reaction. These were then diluted, centrifuged and decanted as before.
Stage 2. Consisted of additional cleaning due to the high organic nature of the samples. In a similar process as before, we added 5mL of 5% HNO3 to the samples, heated to increase the reaction speed. This was allowed to sit and react until completion. The samples were then centrifuged at 2000 rpm for 20 minutes. The samples were decanted, filled with water and centrifuged again at 1200 rpm for 20 minutes. This process repeated for three times.
Stage 3. Consisted of sequential sieving and capturing of samples at 100 μm, 50 μm, 25 μm, 10 μm and 5 μm sieves. All size fractions of the sample were captured, and placed in corresponding 15mL test tubes.
Stage 4. Consisted of heavy liquid separation using sodium polytungstate solution. This solution was brought to a density of 2.17g/cm3 and 5 mL was added to each sample and size fraction. Samples were then centrifuged at 2500 rpm for 20 minutes. Floating material and less dense than 2.17g/cm3 was collected and separated from the more dense material. All samples were then filled with water and centrifuged at 2000 rpm for 15 minutes and liquid was pipetted off. This process of diluting the sodium polytungstate by filling with water, centrifuging and decanting was repeated 7 times for the lighter material and 5 times for the denser material.
Results
After processing the samples according to the Henderson’s Preparation of lake sediments for diatom oxygen-isotope analysismethod, the samples were still contaminated with near density contaminants that were not diatoms. Through SEM (Scanning Electron Microscope) analysis we determined that near density contaminant levels were the predominant material within all samples after heavy liquid separation.
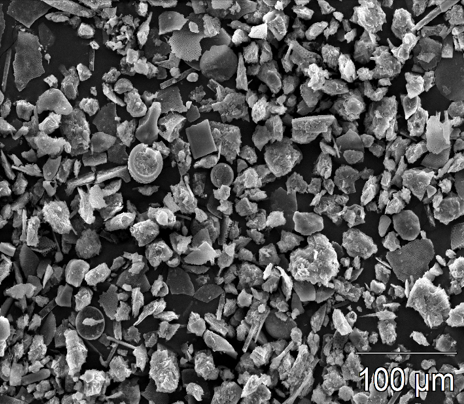
Figure 1: SEM screen capture showing a representative sample of post heavy liquid separation condition of samples. There are sponge spicules (elongate spires), diatoms (flat disc-like structures and thimble structure), tephra (angular clasts with ‘shedding’ features), feldspars (rounded clasts without shedding features), and reprecipitated quartz (unusually flat geometric clast).
Diatom Distribution and Species
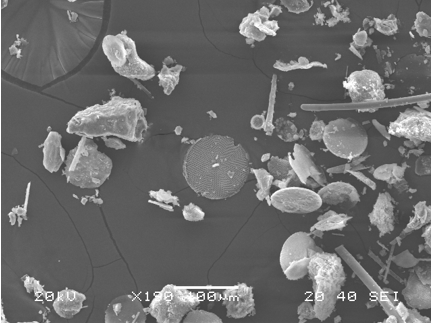
Figure 2: SEM screen capture of sample (87.45-87.46 mbsf) size fraction (100μm-50μm), showing a representative sample of this size fraction containing diatoms (center of picture), sponge spicules (elongate spires), and devitrifying glass (tephra) as angular fragments.
Thalassiosira Torokina Brady were a prevalent species throughout all samples of size fraction <100μm to >10μm in which the samples only produced partial diatom frustules. Typically the diatoms were clay encased as due to their disc-like structure. Diatoms were present throughout all samples of size fraction <100μm. However, the amount of contamination prevents us from proceeding to the next part of the process and undergo mass spectroscopy analysis of these samples to obtain the δ18O value. These samples contaminants through EDS analysis corresponded to what we interpreted as sponge spicules, volcanic tephra, feldspars, and localized reprecipitated quartz. From EDS data most coatings of the diatom frustules contained (K, Al, Mg, and Fe) which we interpreted as clay coatings over these diatom samples. We were able to relatively confirm diatom presence through typical structure and EDS data Figure 3. Full diatoms ranged in size fraction throughout the selected samples. Typically 100μm-50μm produced the largest full diatoms although this size fraction had the least concentration of diatoms. 50μm-25μm provided the richest sample of diatoms in both concentration per unit area as well as diatom species. The trend however as from the oldest section of core (86.60-86.61 mbsf) has an increasing diversity of diatom species and concentration. The variety of diatom species in the younger sections of core show 4 different notable diatom species as compared to 2 in the 86.60 mbsf section of core. The 25μm-10μm size fraction of core predominantly contains diatom fragments and very few whole diatoms. The relative concentration of diatom material however is higher than that of the 50μm-25μm size fraction as much of the tephra, spicule and feldspar contaminant is not present. There are clays present in this size fraction and that makes up most of the contaminant at this size. The size fraction of <10μm contains highly broken diatom fragments and predominantly platy clays. Occasional sponge spicules are present as well however the bulk of the silica at this size fraction are the broken diatom frustules.
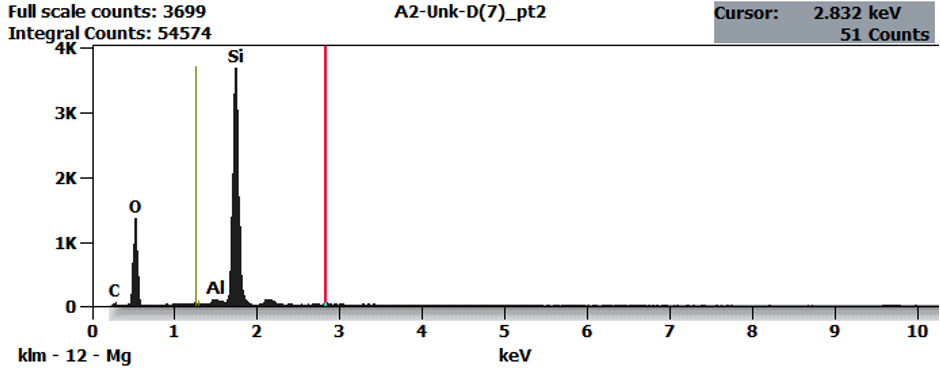
Figure 3: EDS elemental data on circular diatom from Figure 1. Showing a primary composition of Silicon and Oxygen. Carbon and Aluminum are present due to the mounting of the sample, using a carbon film atop an aluminum stud. Therefore those elements are shown within the figure however do not represent the elemental analysis of the sample and instead are background influences.
Sponge Spicule Presence
Sponge spicules are comprised of biogenic silica similar to diatoms and thus through density separation were captured alongside diatom frustules from that process. The spicules also occur in sections in which they are larger than the size fraction of the sieve due to having a spire structure and thus contaminated the samples. EDS data from elongate features of Figure 4 confirm the identity that these structures are biogenic silica spicules.
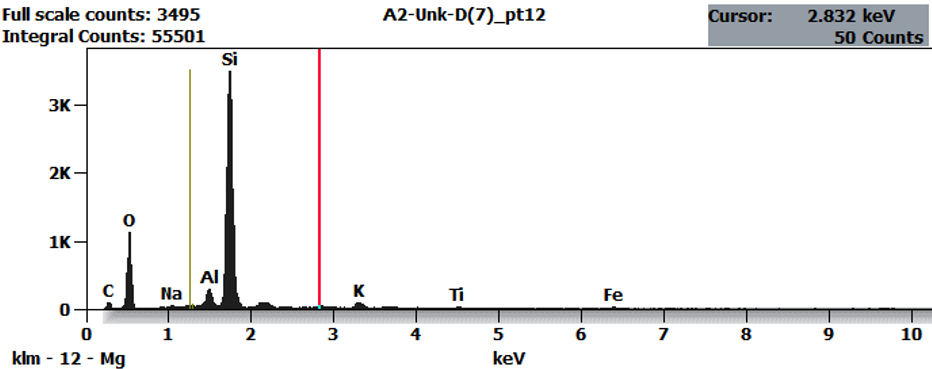
Figure 4: Elemental composition derived from area defined EDS data. Confirming primary composition of Silica and Oxygen with background Carbon and Aluminum. Due to area defined EDS values, clays were present which provided the Potassium, Sodium, Titanium and Iron on the elemental analysis.
Sponge spicules are determined as contaminants as it is not determined if the spicules form in equilibrium in the water and thus if these went through mass spectroscopy would provide error in the δ18O value of the sample. Sponge spicules were a relatively minor component of contamination of the sample as per 2500 μm2 of sample only contained ~7-8 spicules with combined area of 125μm2 of sample. The prevalence of the sponge spicule was throughout all samples however the relative concentration remained the same if not slightly higher as the sediments were younger. The spicules in the younger sediments were as well in better condition and more intact than the older sediments. This could be from diagenesis in differential weathering of the older spicules, or could be representative of the dissolved silica supply in which the younger sediments of this section of core has more abundant DSi level and thus could produce stronger and more resistant spicules.
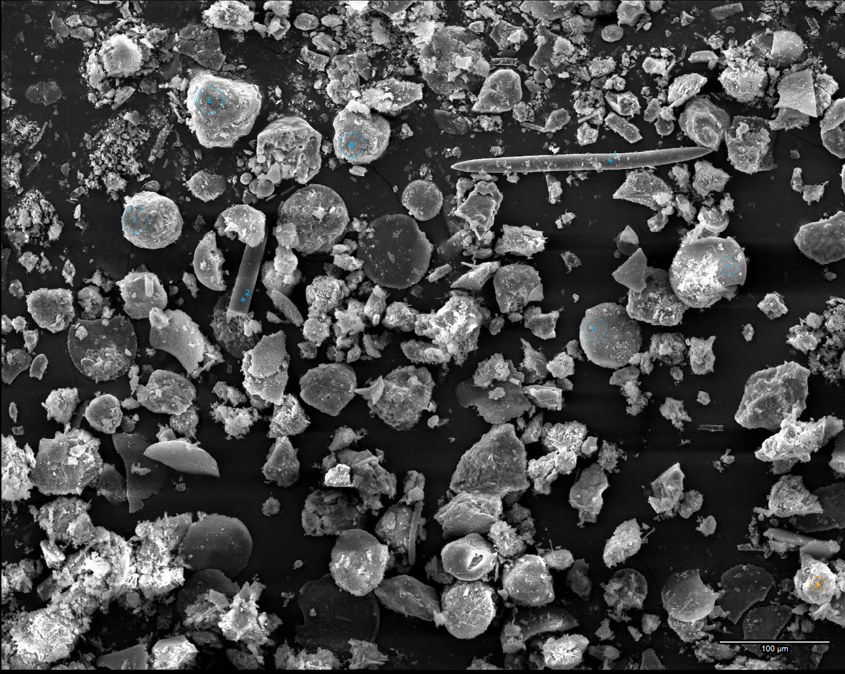
Feldspar Presence
Figure 5: SEM screen capture of sample B3 (87.45-87.46 mbsf) size fraction (50μm-25μm). Noted within the screen capture are EDS datapoints of notable structures/materials within the sample. Points 1-2 are sponge spicules, 3-4 on diatoms, 5-6 and 8 are on non-tephra contaminant. Point 7 is interpreted as tephra.

Figure 6: EDS data on noted points (5,6,and 8) from Figure 5. This data has been normalized for aluminum background interference to accurately assess the likely composition and structure of these non-diatom materials. These points are interpreted as feldspar, specifically potassium feldspar composition from a 1:1 ration of Potassium to Aluminum, and high silica content. Noted accessory elements such as Magnesium, Sodium, Titanium and Iron possibly represent hydration of this feldspar in gradation into clay such as Ilmenite.
Feldspars are located within most samples and all size fractions >10μm. We have interpreted these materials as feldspars from their high Potassium content and above background Aluminum content. As well as an approximate 1:1 ratio of Potassium to Aluminum indicative of an orthoclase (KAlSi3O8) or other potassium feldspar.
Size fraction distribution of feldspar clasts are typically above the <10μm size fraction. However, the feldspar concentration per unit area increases as the size fraction increases. With the most abundant amount of feldspar in the >100μm section. As the size fraction decreases, the amount of weathering feldspars also increase which is to be expected of hydration of feldspars and their correlative size distribution. The feldspar distribution amongst this section of core samples is notable. For the relative concentration of feldspars in the oldest sediment from this diatomite layer (86.60-86.61 mbsf) is the largest in all size fractions of feldspars and decrease in concentration with the layers above. This shows a decrease in feldspar deposition as this “super-interglacial” proceeded. The roundness distribution of the feldspar clasts increase as size fraction decreases. This is possible from clay weathering of the clasts to produce more rounded shapes or that these are older feldspar clasts and have been reworked more times than the more angular clasts.
Overall distribution of these feldspar clasts comprise of the second largest contaminant within these diatomite samples. They comprise 16% by area (ImageJ Analysis) of the contaminant in the 50μm-25μm size fraction of samples.
Accessory elements such as Sodium, Titanium, Magnesium and Iron are likely from hydration of the feldspar and breakdown into subsequent clays. Clays that would incorporate Titanium which would likely be Ilmenite or Illite. These are interpreted as feldspars and not clays due to the roundness of the material, (clays would present a more platy structure even when flocculated) as well as size of coherent clasts. Within all samples, clays were noticed either statically attached to tephra, diatoms or spicules as well as free clay platelets. It is possible that the breakdown of these feldspars are providing in situ clay contamination in these samples as well as clay contamination through diagenesis of these samples.
Precipitated Quartz Presence
In Figure 1 near the large thimble-like resting spore is an unusually flat geometric material.
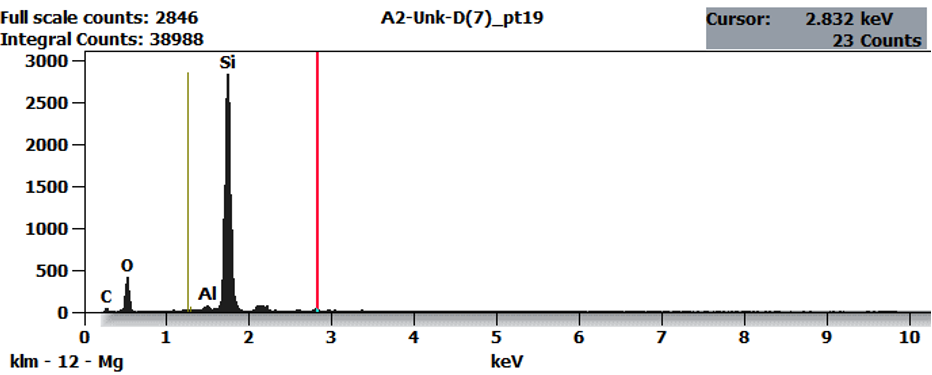
Figure 7: This graph is the EDS data on point 19 in Figure 1. Showing a primary composition of silicon and oxygen with background levels of aluminum and carbon. This would lead us to interpret this piece as likely quartz.
This piece is notably unusual as though it has a composition of silicon and oxygen which would lead us to interpret this material as quartz, there is no devitrification upon this grain, nor does it show any rounding (evidence of reworking). This leads us to interpret this material as precipitated quartz from dissolved silica in this sample. The age of this quartz seems to be younger than that of the other silica contaminants due to lack of roundness and lack of devitrification. The distribution of these quartz fragments are typically within the larger size fractions of the samples. Found only in the >100μm , 100μm-50μm and 50μm-25μm size fractions. As well these quartz fragments are only found in the lower portion of this diatomite section.
Tephra Presence
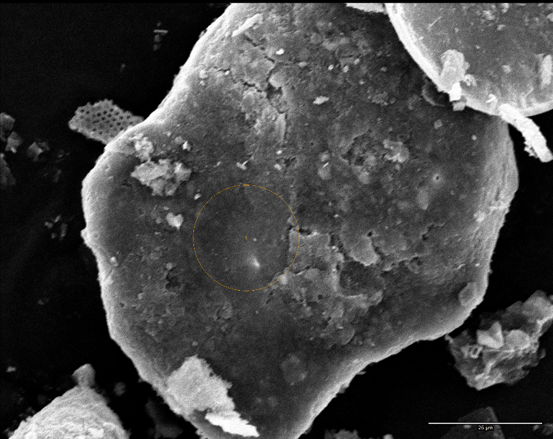
Figure 8: This SEM image shows a representative single grain that is interpreted to be devitrifying volcanic silica glass (tephra). EDS data upon this sample shows silica composition of the main clast as well as the ‘flakes’ that are weathering from the product, leading us to interpret this as devitrifying glass.
This by far is the largest contaminant of the sample. In total tephra comprises approximately 73% of the total contaminant in the set of samples. Although the tephra clasts typically are found in the larger size fractions >100μm and 100μm-50μm some pieces of broken tephra have been seen in the smaller size fractions. In the 25μm-10μm section of the core, full tephra clasts are not typical. Instead crushed/flaked tephra pieces are found instead. This could result from the mounting process in which the weaker tephra pieces were broken during mounting, or could result from the collection of previously broken tephra flakes. This changes in the <10μm size fractions however clays make up the predominant contaminant. Many of these grains remain angular with slight rounding on the smaller size fraction of the tephra.
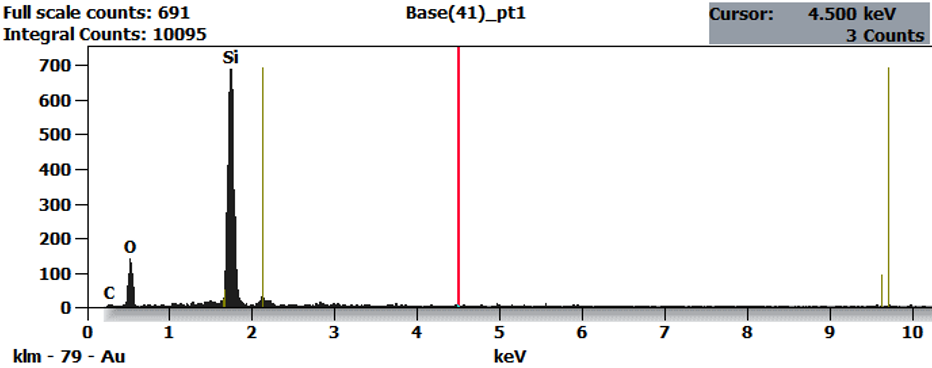
Figure 9: Shows the chemical composition of the angular devitrifying grain with a primary composition of silicon and oxygen with background carbon from the carbon film. This EDS data point is an average over the circled area in Figure 7. Thus taking into account the flakes upon the grain. Noting that there is no potassium, magnesium, iron, or titanium within this area of analysis we can conclude that those flakes are not clay minerals upon this mineral grain.
From the EDS data we can heavily imply that these are indeed tephra particles as the primary composition if that of silicon and oxygen. This would imply that these are indeed quartz grains, however the presence of ‘flakes’ show that this is likely devitrifying volcanic glass as typical quartz mineral grains do not show ‘flaking’ at this level and only short-ordered glass would produce such an image. The sheer amount volcanic glass within this section is likely to be seen in this stage as it has an identical density to that of diatoms, as they both are composed of silica. Much of these tephra are devitrified heavily, which would imply that these are reworked sediments from a previous eruption and do not correspond to this period in time. Although there is likely a diagenesis factor that could affect the rate of devitrification, we interpreted that these would be reworked sediments that have been deposited here in this diatomite section. This contaminant is the most problematic of the listed contaminants so far, as it has an identical density to that of diatom frustules and cannot be removed by differential charge or other electrical means.
Discussion
The large amount of contaminants for these samples provide a barrier to gather δ18O values of the diatom frustules. However presents us with a unique challenge as to interpret the processes of deposition for these samples and their implications for the paleoenvironment of the Pliocene-Pleistocene transition. The amount of near density contaminants and presence of silica contaminants would change the isotopic signature of these diatom frustule samples. As much of the contaminants are tephra which is identical composition to the diatom frustules, this prevents these samples from being able to be analyzed under mass spectroscopy until the contaminant is removed. This provides a unique challenge as density would not be sufficient to separate the contaminants from the sample. This leaves us with interpretations as to the depositional environment and processes which transported and deposited these contaminants into these samples.
Sponge Spicule Implications
Sponges are benthic organisms and from these spicules were clearly present that this time in the vicinity of the AND-1B drill site. It is likely that there was little to no transport of the sponge spicules to the AND-1B drill site. As this time is interpreted as open ocean conditions it is most likely that these benthic sponges were present at the drill site. It is not fully known if sponge spicules form with isotopic equilibrium of the water. If sponge spicules did form within equilibrium of the seawater then that signature could be used as a paleo environmental proxy for paleoclimate conditions. Even if that were the case that sponge spicules did form without any oxygen fractionation, they would not necessarily be representative of the same waters that the Antarctic diatoms are representing. As they are benthic organisms which at times have the ice sheet grounding line very close could be representing the lower brines of the ice shelf and Antarctic bottom waters. This section of the water column would not be represented by the near surface photosynthetic diatoms and thus contaminate the isotopic signal of this sample selection. The increase quality of spicule difference as the sediments progress younger implies a greater amount of dissolved silica that penetrates further into the water column as time progressed during this “super-interglacial”. Another consideration could be that simply the older sediments were exposed to further diagenesis or reworking than the younger sediments and that is the reason for an increase in broken and low quality spicules in the lower section.
Feldspar Clast Implications
The presence of feldspars within this sample are notable as they are not purely silica materials and are possibly the main clay mineral sources for the capping mudstone lithology above this diatomite unit. These feldspar clasts within the sample are heavily rounded and undergoing hydration, weathering into clay minerals. This is interpreted from the amount of accessory minerals present in the EDS data done on feldspar grains. The capping unit is a highly laminated mudstone. If this mudstone contained a higher concentration of titanium rich clays, then the feldspars in this section, or the feldspar source could be the primary source of the clay minerals that comprise that mudstone.
This could imply that these feldspars were reworked sections of previous arkosic units, however it is more likely these are ice-rafted or glacially transported debris. Due to the presence of diatomite it is interpreted this time as an open ocean condition. Thus overhead glacimarine transport is not likely as a transport mechanism to deposit feldspar grains at the AND-1B drill site. The bedrock along the continental coast near the AND-1B drill site are the Granite Harbour Intrusives (granodiorite and hornblende granite)10. This is the likely source of the original potassium feldspar grains. A possible interpretation of a transport mechanism would be that these grains were deposited by turbulent deep-water currents. These currents could produce the heavy rounding of these feldspar grains from their continental origin to that of the AND-1B drill site. According to Naish et al 2008, it is interpreted that the grounding line was near the continental coast, which implies that the most likely transport mechanism would be glacial ice rafting. This transport mechanism would be able to explain the roundness of the feldspar grains as well as a physical transport method to the drill site. The relative concentration of the feldspars as they decrease in concentration as the unit becomes younger would fit with the interpretation of ice rafted debris. During the Pliocene-Pleistocene transition as the ice sheet retreated to the coastline it was closer than it was during the peak of this Pleistocene age “super-interglacial” thus a greater amount of ice rafted debris would be deposited on the site, as the further the grounding line moved away from the drill site, less and less ice rafted debris would be deposited.
Precipitated Quartz Implications
The presence of precipitated quartz is contentious. It is possible for these grains to be directly volcanic related, granodiorite related or to be precipitated quartz. It is unlikely to be directly volcanically related as there is no presence of a recent volcanic eruption at this site during this time. As well as the lack of weathering upon this quartz would imply a younger origin of the clast. If these clasts were granodiorite related, we would expect to as well see signs of rounding from glacial processes, similar to the feldspar clasts. That possibly could explain the unique angular geometric shape of this grain however if this quartz indeed was an intrusive. Due to the lack of weathering however, we interpreted this material as precipitated quartz. The lack of weathering implies a younger origin of this material than the deposition of the sediment. As this is a silica rich sediment that has gone through levels of diagenesis, it is quite possible that an amount of dissolved silica would precipitate as a new quartz grain within the sediment. This does not however explain the unique flatness to the grain as all other materials within this sample are weathered, angular and irregular polygons. While these grains contain faces that are flat and possess a rectangular prism polygonal quality. This seems to be evidence for diagenesis of these samples which questions their validity for their δ18O values, as the diatom frustules could have equilibrated with the oxygen of the water within that saturated sediment. Which would then change their isotopic signature to not represent the water of formation of that frustule.
Tephra Implications
As the largest contaminant within the set of samples this has the largest implications on the study. This is the primary barrier to progress this study to be able to obtain the δ18O values from the diatom frustules. The presence of silica tephra prevents any further refining of density separation as diatom frustules and tephra would have an identical density. Removing this type of contaminant would be the largest hurdle to purify these samples to undergo mass spectroscopy.
The source of the tephra is important as to the environment of deposition for this study. The source of the tephra has a few possibilities. It is possible to be a product of a volcanic event during this “super-interglacial” and as the most resistant mineral of mafic mineral sets would be the only remnant from this limited event. There is no other evidence of nearby volcanism during this period in the Ross sea. There could be other mafic minerals present within the sample but were separated out during the heavy liquid separation stage of preparation.
We posit however that the source of this tephra contaminant is reworked volcanic sediments from the 1.65 Ma age volcanic sandstone. Due to the presence of devitrification we can interpret the silica contaminant as volcanic glass. Due to the amount of devitrification we can imply that the sediment has been reworked. Volcanic glass does not form in equilibrium with the water, which would clearly skew the δ18O value of the sample. As well this silica is a possible source of the high dissolved silica water that formed the precipitated quartz. Which as a process of diagenesis could have possibly altered the δ18O of the diatoms in this section of core.
Diatom δ18O Implications
As we were unable to purify the sample to reach the mass spectroscopy stage of the study, the implications of glacial cyclicity from δ18O values are unknown. Likely problems with the δ18O values of the diatom frustules would as well be the aforementioned likely diagenesis within these sediments and their effects on the δ18O values. If these diatoms equilibrated with the new DSi rich pore water the δ18O signal would be skewed and not be representative of the paleoclimate.
Future Work and Research
In order to further these samples along the purification process, all contaminants must be removed. Sponge Spicules and feldspar clasts could be removed through further amounts of heavy liquid separation at more precise sodium polytungstate densities. Further refinement through heavy liquid separation could reduce the feldspar contamination, and through further cycles of sieving, the large sponge spicules contamination would be reduced. The tephra contamination however is a more difficult challenge. A possible procedure to remove the tephra from this sample would be following Lamb et al., 2007 procedure on removing tephra from lake diatom sediments. This procedure however would have to be reworked for this core as the Lamb procedure has only worked on samples with <5% contamination11. This was partially done through geochemical analysis of the tephra contaminant and isolation of the contaminant source. Once that source contaminant was analyzed with mass spectroscopy that δ18O value of the diatom sample was normalized with that δ18O value relative to the percentage of contaminant. In order to further the research for this study, a δ18O value of the 1.65 Ma volcanic sandstone and normalization for these diatom samples would be able to produce a δ18O value representative of only the diatom frustules.If the samples were able to be purified to <5% contamination, the diatoms could then undergo mass spectroscopy analysis and thus be able to be quantified for paleoclimate interpretations.
Acknowledments
The ANDRILL studies were supported by the US National Science Foundation, the NZ Royal Society of New Zealand, Marsden Fund, and the Italian Antarctic Research Programme, the German Research Foundation, and the Alfred Wegener Institute for Polar and Marine Research. Special thanks to Jessica Mckay, Sarah Shapley, Jake Bergeron and Monica Burrel for assistance in the lab.
References
1. Naish, T. R.; Powell, R. D.; Barrett, Peter J.; Levy, R. H.; Henrys, S.; Wilson, G. S.; Krissek, L. A.; Niessen, F.; Pompilio, M.; Ross, J.; Scherer, R.; Talarico, F.; Pyne, A. R.; and ANDRILL-MIS Science Team, “Late Cenozoic Climate History of the Ross Embayment from the AND-1B Drill Hole: Culmination of Three Decades of Antarctic Margin Drilling” (2008). ANDRILL Research and Publications. Paper 26. http://digitalcommons.unl.edu/andrillrespub/26
2. McKay, R.; Powell, R.D.; Naish, T. R.; “Antarctic and Southern Ocean influences on Late Pliocene global cooling” (2012). PNAS. Vol. 109, No. 17, www.pnas.org/cgi/doi/10.1073/pnas.1112248109
3. Meyer, H., et al., Oxygen isotope composition of diatoms as Late Holocene climate proxy at Two-Yurts Lake, Central Kamchatka, Russia, Glob. Planet. Change (2014), http://dx.doi.org/10.1016/j.gloplacha.2014.04.008
4. Winter, D., et al., Pliocene–Pleistocene diatom biostratigraphy of nearshore Antarctica from the AND-1B drillcore, McMurdo Sound, Glob. Planet. Change (2010), doi: 10.1016/j.gloplacha.2010.04.004
5. Powell, R. D.; Naish, T. R.; Krissek, L. A.; Browne, G. H.; Carter, L.; Cowan, E. A.; Dunbar, Gavin B.; McKay, R. M.; Wilch, T.; and ANDRILL-MIS Science Team, “Antarctic Ice Sheet dynamics through the Neogene from evidence in the ANDRILL–McMurdo Ice Shelf Project drillcore (AND-1B)” (2007). ANDRILL Research and Publications. Paper 38. http://digitalcommons.unl.edu/andrillrespub/38
6. Pollard, David and DeConto, Robert M., “Modeling West Antarctic ice sheet growth and collapse through the past five million years” (2009). ANDRILL Research and Publications. Paper 35. http://digitalcommons.unl.edu/andrillrespub/35
7.
8. Lisiecki, L. E., and M. E. Raymo (2005), A Pliocene-Pleistocene stack of 57 globally distributed benthic d 18O records, Paleoceanography, 20, PA1003, doi:10.1029/2004PA001071.
9. Bailey, H. L., Henderson, A. C. G., Sloane, H. J., Snelling, A., Leng, M. J. and Kaufman, D. S. (2014), The effect of species on lacustrine δ18Odiatom and its implications for palaeoenvironmental reconstructions. J. Quaternary Sci., 29: 393–400. doi:10.1002/jqs.2711
10. Passchier, S.; Hauptvogel, D.; “Early–Middle Miocene (17–14 Ma) Antarctic ice dynamics reconstructed from the heavy mineral provenance in the AND-2A drill core, Ross Sea, Antarctica” Glob. Planet. Change (2012). doi:10.1016/j.gloplacha.2011.11.003
11. Lamb, A. L.; Brewer, T. S.; Leng, M. J.; “A geochemical method for removing the effect of tephra on lake diatom oxygen isotope records.” (2007). J Paleolimnol (2007) 37:499–516, DOI 10.1007/s10933-006-9034-5